This week’s BEACON Researchers at Work blog post is by University of Texas at Austin graduate student Chelsea Weitekamp.
An unlucky vampire bat returning to roost at night with an empty belly can solicit help from a roost-mate to avoid starvation. A young Florida scrub-jay will forgo years of breeding opportunities to help rear their siblings and defend their parents’ territory. A subordinate lance-tailed manakin will perform an outrageous dance to help his dominant partner secure a female and will get nothing immediately obvious in return.
Decades of work have provided us with a solid understanding of how such cooperative behaviors evolved, but this doesn’t necessarily answer the question of what is going on in their in minds, in their brains. It can help, though. For example, in vampire bats, cooperative food sharing through regurgitation is more likely if the hungry bat had previously shared a meal of her own, and if there has been more allogrooming between the two. This implies the involvement of memory and individual recognition.
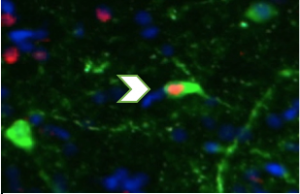
The arrow shows an ‘active’ dopamine neuron, a method I use in A. burtoni to investigate the role of the reward system in cooperative behavior.
I am interested in what underlies decision-making in these examples of cooperative behavior. Going back to the bats, we can visit the extremes of anthropomorphism, and think, “how sweet, that vampire bat is compassionate, helping out her dearest friend,” or we can call on Descartes, and regard these behaviors as a means by which the machine-bat continues to survive, a reflex, perhaps, but certainly nothing conscious. Fortunately, modern techniques can help to shed some light on the issue. We can start by asking which brain regions are active during the cooperative behavior. So, if regions known to be involved in emotional processing are activated, do we have evidence of “higher cognitive processing”? It’s a step. What if we determine the reward system to be a crucial component in determining whether or not the female bat shares her meal? What if oxytocin, the so-called “love hormone” is released when she shares? Or not? Where does this get us? Closer.
The types of cooperative behavior in which I am interested involve evidence of a cognitive component. While cooperation can often be achieved through simple rules, there are many cases in which behaving cooperatively requires learning, memory, and individual recognition, as in the bats. An interesting question arises – is cooperation really a special phenomenon? Cooperation exists, of course, but it can be defined within very narrow or very wide terms, depending who you ask. So, does a circuit of sorts exist in the brain, common across different types of cooperative behavior and common across species? Is it realistic to expect a similar neural basis for behaviors that are so difficult just to define?
To address these questions, I study fish. Since diving into the world of fishes, I have been amazed by the behavioral complexity of which fish are capable. And they happen to be particularly great models for studying cooperation. For example, groupers sometimes hunt collaboratively with moray eels, using referential gestures to oust hidden prey. Pretty impressive for species considered by some to not even be wildlife.
Cleaning wrasse and their clients, though, may take the cake for impressive behaviors. Cleaner wrasse set up shop along the coral reef and ‘clients’ pay them visits to have ectoparasites and dead tissue removed. Cleaners actually prefer client mucus which is costly to cleaners, and hence, by suppressing their preference, cooperation arises out of conflict. Cleaners still try to get away with stealing mucus when they can. Redouan Bshary and others have showed that cleaners will cooperate more when being observed by by-stander clients. They also tend to be more cooperative with predatory clients, a good choice. We recently started a collaboration with Dr. Bshary to try to get at the neural basis of some of these nuanced behaviors.
Cichlids offer some competition, as far as impressive behaviors go. Of course there isn’t enough room here to discuss all the ways in which cichlids are smarter than some humans, but I’ll start. The Hofmann lab studies an African cichlid, Astatotilapia burtoni. Males of this species are highly perceptive of their social environment, and also respond differently depending who is watching. Neighboring territorial males will also cooperate by forming defense coalitions against invading wanna-be males trying to steal their space. I use these paradigms to try to get at what is going on in the brain when the males are processing these different stimuli and making decisions. I look at what brain regions are involved, and at what gene pathways within those brain regions may be regulating behavior.
Of course, we’ll never really know what these fish are thinking. Thomas Nagel offers some insight on the matter, in considering what it is like to be a bat (yes, more bats): “Our own experience provides the basic material for our imagination, whose range is therefore limited. It will not help to try to imagine that one has webbing on one’s arms, which enables one to fly around at dusk and dawn catching insects in one’s mouth; that one has very poor vision, and perceives the surrounding world by a system of reflected high-frequency sound signals; and that one spends the day hanging upside down by one’s feet in an attic. In so far as I can imagine this (which is not very far), it tells me only what it would be like for me to behave as a bat behaves. But that is not the question. I want to know what it is like for a bat to be a bat.”
For more information about Chelsea’s work, you can contact her at chelseaweitekamp at gmail dot com.