This week’s BEACON Researchers at Work blog post is by University of Idaho graduate student Joshua Sukeena.
The sense of vision is mediated by a specialized projection of the central nervous system located in the back of the eye, the retina. The retina is a highly conserved structure consisting of cellular and synaptic layers that establish physiological pathways capable of converting light stimulus from our environment into a perceivable image (figure 1). This is possible because of the specialized circuits that detect different aspects of vision such as color, edges, and movement. Light first stimulates photoreceptors at the back of the retina, which propagate this information to innernerons that modulate this signal before the retinal ganglion cells send this information to the brain.
Our lab is interested in how this structure has evolved, and we operate with a focus on the visual system of teleost fish. The visual system observed in teleost fish is interesting because of its ability to regenerate damaged neurons, maintain constant neurogenesis throughout their lifespan, detect a wide range of light, and adapt to novel visual environments, and they contain an increased number of retinal cell types. We hypothesize that the specialization and unique traits that are observed in the teleost visual system was influenced by the Teleost Genome Duplication (TGD). This was a large scale genetic rearrangement event that took place roughly 350 million years ago, providing the the teleost lineage with a duplicated genome that resulted in an explosion of diversity.
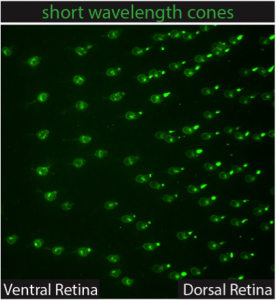
Figure 2. Spotted Gar retina with antibody to short wavelength cone opsin. A clear gradient was observed in cellular density between dorsal and ventral regions of the retina. Density between the two regions was significant (p= 2.07×10-41).
As a first step to understanding the role of TGD on the evolution of the visual system, we have characterized a basal model, the spotted gar, which diverged in lineage prior to the TGD. This gave us the unique opportunity to study a retina that evolved under similar environmental pressures, but with only a single genome. Consistent with our hypothesis, we found the retina of the spotted gar to have conserved structure and cell types to that of the teleost, but some key differences were observed. The teleost retina is well known to have a highly ordered photoreceptor mosaic. The retina of the spotted gar was organized, but not to the level and consistency that was observed in teleost. The spotted gar retina also contains gradients of cellular density throughout the eye; a trait seen in mammals but not in teleost fish (figure 2). The spotted gar retina also contained thinner cellular layers, supporting the hypothesis that teleost retinas contain more retinal cell types overall. Through this characterization, we have been able to identify and label major cell popluations in the retina, as well as many different individual cell populations including cones and amacrine cells (figure 3). This will be beneficial to the our project going forward, as well as future research involving the spotted gar retina.
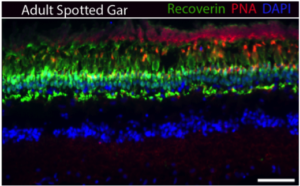
Figure 3. Spotted Gar retina section stained with Recoverin (photoreceptors), PNA (cone pedicles), and DAPI (nuclear stain). Retina structure is conserved compared to zebrafish and mammals. Scale bar is 20 um.
Next we hope to assay the function of paralogs in the zebrafish retina. The zebrafish is a member of the teleost lineage, and is a common model for eye research. When gene duplication occurs, there are three possible outcomes. Neofunctionalization occurs when the duplicated gene develops a completely novel function(s). Pseudogenization occurs when the gene becomes obsolete and its expression is lost. Subfunctionalization occurs where the two genes each retain a “subset” of the original gene function and are possibly relegated to expression in a specific tissue or cell type.
We will initially target two cell adhesion molecule families, the sidekick (SDK) and Down syndrome cell adhesion molecule (DSCAM) families, both essential in the organization of the retina. These are ideal candidates because the zebrafish genome contains twice as many copies as the spotted gar (and mouse). We will use genomic informatics and molecular approaches to assay whether both copies of the duplicated genes have been retained in teleost retina.
We will begin by performing in situ hybridization assays on the paralogs of our target genes. This will be done across development for the zebrafish to determine whether expression patterns have diverged. We will utilize developmental assays performed with the use of two different gene knockdown techniques. Morpholino oligonucleotides are small miRNA sequences that target specific mRNA sequences and reduce translation of these transcripts. The CRISPR/Cas9 technique was only recently discovered, but provides a unique way to target specific sequences in the genome directly. This will allow us to assay if the respective function of each gene has diverged compared to its paralog and therefore test if neofunctionalization has occurred. We predict the genome duplication and associated increase in genetic material could have enhanced the potential of the teleost lineage to develop novel visual circuitry. Future plans include adapting a place preference test in order to look at visual acuity in the mutant fish we develop.
My path to a PhD program has not been traditional. I began at The College of Idaho in pursuit of a degree in history and education. However, spending time in a cognitive research lab drastically changed my outlook for my future. This experience inspired me, but I knew that my biggest interests were at the molecular level; specifically in the field of genetics. I joined the University of Idaho in the lab of Dr. Peter Fuerst in the fall of 2013. Having grown up in Idaho, I have become aware of the problems facing scientific education. It is a state that ranks near the bottom of the country in number of students that go on to get a technical degree, college degree, or post-graduation degree. I am determined to make sure these statistics do not persist. I know that a doctoral degree will not only lead to an opportunity to conduct research and contribute the scientific community as a whole, but also put me in a position
to make a significant impact on the way my community looks at science and education as tools for improvement. Idaho has abundant environmental resources and opportunity for young scientists. I have a dream of establishing an educational program which allows children to travel and learn about different disciplines of science all over our state from geology to wildlife science and microbiology. I believe in a future where scientific inquiry and research understanding is a commonplace in our educational system; allowing it to persist and lead to a more stable future.
For more information about Joshua’s work, you can contact him at jmsukeena at gmail dot com.