This week’s BEACON Researchers at Work post is by University of Washington postdoc Brian Connelly.
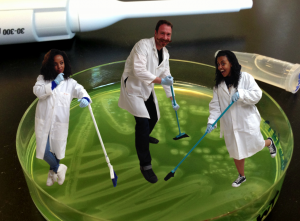
Just another day in the lab. Making plates with Belen Mesele (l) and Helen Abera (r), two of the people working on the project with me. Our wild-type cooperator strains produce beautiful blue-green colonies due to the production of pyocyanin, another behavior regulated by quorum sensing.
Evolutionary biologists often talk like economists, particularly when the topic is cooperation. Instead of dollars, euros, or pounds, the universal currency in evolution is fitness. A species that cooperates cannot survive when competing against a non-cooperative opponent unless the fitness benefits provided by cooperation, such as those resulting from greater access to resources, outweigh the costs. To make matters more complicated, cooperative benefits often take the form of “public goods,” which benefit all nearby individuals, whether cooperator or not. This sets the stage for the emergence of “cheaters,” which exploit the cooperation of others without contributing themselves. Despite cooperation seeming at odds with the notion of “survival of the fittest,” we now have a good understanding of how cooperation can persist in the face of cheaters based on the tremendous work of Fischer, Haldane, Hamilton, Price, and those who have since followed. When the costs and benefits are favorable, and when close relatives are more likely to receive those benefits, cooperation can survive and even thrive.
Environments are always changing, and since the environment plays a dominant role in determining the fitness costs and benefits associated with all traits, natural selection may quickly change between favoring cooperation and not. When the balance shifts so that cooperation becomes more costly than beneficial, cooperators risk being driven to extinction by cheaters or other non-cooperators that do not pay those costs. So how can cooperators survive these tough times? The answer is frustratingly simple—by not cooperating. The challenge, though, is in determining when to cooperate and when to be more self-centered. We humans and other primates are—perhaps very arguably—good at estimating whether or not cooperation will benefit ourselves and those with whom we are similar, either genetically or in our beliefs. We are able to do this by integrating a great deal of information about our world and the people in it. But we are not alone in this.
Surprisingly, it turns out that even relatively “simple” bacteria are extremely effective at determining whether or not to cooperate based on the state of their environment and the composition of their population. One of the ways that these bacteria accomplish this is through quorum sensing. With quorum sensing, individuals communicate with each other by releasing and detecting small molecules, which are used as signals. When an individual detects low levels of the signal, it can use this information to assume either that there are too few other cooperators nearby to produce sufficient benefits by cooperating, or that the public good will be flushed out of the environment before it can be used. However, when that individual detects high levels of the signal, it is likely that there are many relatives nearby that would benefit from cooperation. By communicating this way using signals specific to their own species, bacteria use quorum sensing to rapidly adjust their behaviors to maximize their fitness as the environment changes.
Josephine Chandler recently wrote about her fascinating work that addressed how bacteria use quorum sensing to control the production of antibiotics. While she investigated this process as a means of competing with other species, it can also be viewed as a form of cooperation among members of the same species. By using antibiotics to kill off competitors, sometimes self-sacrificially, more resources become available to those that remain. And because species often have resistance to the antibiotics that they produce, those that remain after an antibiotic attack are likely to be close relatives.
The use of antibiotics is just one example of a behavior controlled by quorum sensing. Since its discovery in the early 1970s, quorum sensing has been observed across a wide variety of species. Among the behaviors regulated by quorum sensing, those related to cooperation and other social interactions are perhaps the most prevalent. Because of this, quorum sensing is believed to play a key role in allowing cooperation to persist in ever-changing environments.
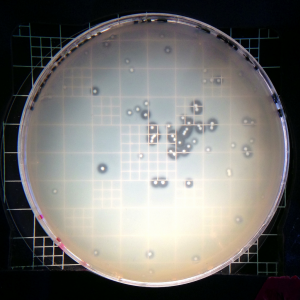
Colonies formed by two of our strains. Through the production of elastase, our cooperators are able to break down the proteins present in this milk agar plate, forming large, clear halos. Our non-cooperator strain does not produce elastase, so it is unable to break down the milk proteins, and a much smaller halo is produced.
Although the connection between quorum sensing and cooperation is now well known, little is understood about how these behaviors became interlinked. To begin addressing this, I am currently working in Ben Kerr’s lab on a number of projects that investigate the co-evolution of cooperation and quorum sensing. To gain a broader picture of this process, we’re pairing microbial experiments with computational and mathematical models. The cooperative behavior we’re focusing on in our study system, Pseudomonas aeruginosa, is the production of the digestive enzyme elastase. When secreted into the environment as a public good, elastase breaks down large proteins into smaller, usable sources of nutrients available to all cells in the surrounding area. In environments where these large and otherwise inaccessible proteins are the main nutrient source, this behavior is extremely beneficial. (Here is a short video demonstrating growth of our bacteria.)
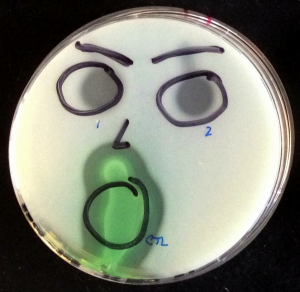
In these environments, where the proteins are a limited source of resource, cooperators do better due to the benefits provided by elastase. We can measure the amount of cooperation occurring within populations by examining the size of the clearing that occurs when extracting and plating the elastase that is produced. Note: faces add no scientific value.
By exposing our populations to different environments over many generations, we are directly observing how communication and cooperation co-evolve. Through these experiments, we are investigating how quorum sensing enables cooperation to be maintained, the types of environments in which this occurs, and the different ways in which this regulation can occur. We hope that through this work, we can gain a greater understanding of the complex social processes that occur in natural ecosystems and in some of the infections that create tremendous health challenges.
For more information about Brian’s work, you can contact him at bdc at bconnelly dot net.