This week’s BEACON Researchers at Work blog post is by University of Washington graduate student Shawn Luttrell.
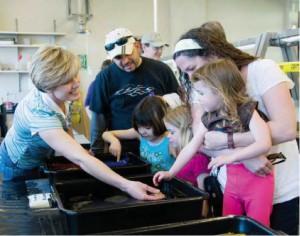
Shawn Luttrell at Friday Harbor Laboratories Open House, showing invertebrates to the public. Photo courtesy of Kathy Ballard.
Regeneration has captured the interest and imagination of people for centuries. Popularized in myths, science fiction, and even horror movies, regeneration of missing and damaged tissue is anything but fictional in the animal kingdom. Nearly every animal phyla contains at least some species that consistently regenerate all or certain tissues and structures. All deuterostome groups, with the possible exception of cephalochordates and xenoturbella, have at least some species with the capacity to regenerate (Sánchez Alvarado, 2000). Numerous chordates, like tunicates, frogs, fish, salamanders, and even humans are able to regenerate to some degree. Every extant class of echinoderms have been reported to regenerate, while some species of hemichordates, which are a sister group to the echinoderms, are capable of regenerating all body structures (see figure below; Candia Carnevali at al., 2009; Rychel and Swalla, 2008; Humphreys et al., 2010). When and in what animal lineage did the ability to regenerate first evolve? Was regeneration a stem metazoan trait that was subsequently lost or reduced in numerous taxa or has regeneration evolved independently several times across the metazoans? These questions, which remain unanswered after years of study, fascinate me and form the foundation of my Ph.D. graduate thesis research.
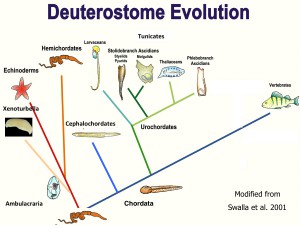
Deuterostome phylogeny showing the hemichordates in a sister group to the echinoderms. Image modified from Swalla et al., 2001.
Understanding the morphological and genetic mechanisms for regeneration may yield clues to unlocking regeneration in animals with limited or no regenerative abilities, like humans. Millions of people suffer from neurodegenerative diseases, spinal cord injuries, and limb amputations. Furthermore, aging and age related diseases affect every person on the planet. Regeneration may slow the aging process and stem cells present feasible ways to combat a multitude of diseases and injuries. If regeneration is a stem deuterostome trait, it is likely that humans possess many, if not all, of the genetic switches controlling regeneration, but those switches have been modified or inactivated in some way over evolutionary time. It may therefore be possible to re-activate those pathways in humans using genetic models made from animals with extensive regenerative capabilities.
To help elucidate the origin of regeneration, I am working with Dr. Billie J. Swalla at the University of Washington to identify the genetic underpinnings controlling regeneration in the hemichordate, Ptychodera flava, which is a basal deuterostome and capable of regenerating all anterior and posterior structures when bisected (Rychel and Swalla, 2008; Humphreys et al., 2010). We are collaborating with Dr. Alejandro Sánchez Alvarado of the Stowers Institute for Medical Research to sequence, assemble, and analyze the transcriptome of P. flava. This is part of an overarching transcriptome project, across numerous animal phyla, to determine whether there is a common gene or set of genes expressed across all species during regeneration. If the same genetic mechanisms are used, this would supply data to support the theory that regenerative powers evolved once from a common ancestor to all animals studied. If the data shows little to no overlapping expression in genes of interest, this may support the hypothesis of multiple, independent, evolutionary nodes of regeneration. As it is much easier evolutionary to lose a trait rather than gain, we believe the data will support a single line of descent.
I am also working to characterize expression patterns of neural genes and then map that to morphological changes accompanying nervous system regeneration in P. flava to supplement the transcriptome data. As a basal deuterostome with a bona fide central nervous system and remarkable regenerative powers, this animal presents an exciting model to study nervous system regeneration. With this and the transcriptome data, we can directly compare regeneration of the neural tube to early development of the neural tube and ascertain whether regeneration is a morphological and genetic recapitulation of development. Preliminary results suggest regeneration may employ morphological pathways not invoked during early development. If confirmed, this result is exciting because it means there are multiple ways to achieve the same biological structure and demonstrates developmental plasticity during regeneration.
Another component of my thesis work will be to determine the origin and character of stem-like cells employed during hemichordate regeneration. Are these cells multi-potent stem cells with similar genetic expression as vertebrate stem cells or are the cells coming from a population of differentiated cells that have returned to a progenitor cell state and are assigned new cell fates in regenerating tissue? To answer this question, I will use in situ hybridization with known vertebrate stem cell markers, like piwi, vasa, and nanos. Expression of these markers, coupled with functional identity, would support hemichordates having genuine multi-potent stem cells. If basal hemichordates and vertebrates both possess multi-potent stem cells, then the common ancestor of the deuterostomes likely had these cells as well. If, on the other hand, expression and functional relatedness cannot be confirmed, this might suggest that differentiated cells are undergoing cell fate reassignment. In humans, when a cell differentiates into a particular cell fate, the cell’s condition cannot be reversed or altered. If cells are undergoing cell fate reassignment in hemichordates, this will have implications for creating stem cells from differentiated cells in humans by revealing cell signaling pathways necessary for the gain and loss of cell fates.
Though I am in the early stages of my research, I have already obtained exciting preliminary data. I have collected live P. flava and performed various regeneration experiments. I have isolated total RNA at different stages of
regeneration for the transcriptome. Sequencing and assembly is currently underway. I have also fixed numerous animals and I am making sections of the regenerating tissue for staining with particular biomarkers to obtain a clear picture of how and when missing neural structures are elaborated. My research may reveal key evolutionary mechanisms of regeneration in the deuterostomes.
References
Candia Carnevali, M.D. (2006). Regeneration in echinoderms: Repair, regrowth, cloning. Invert Surv J. 3, 64-76.
Humphreys, T., Sasaki, A., Uenishi, G., Taparra, K., Arimoto, A,. Tagawa, K. (2010). Regeneration in the hemichordate Ptychodera flava. Zoolog Sci. 27(2), 91-95.
Rychel, A.L. and Swalla, B.J. (2008). Anterior regeneration in the hemichordate Ptychodera flava. Dev. Dyn. 237(11), 3222-3232.
Sánchez Alvarado, A. (2000). Regeneration in the metazoans: Why does it happen? Bioessays. 22, 578-590.
For more information about Shawn’s work, you can contact her at shawnluttrell at gmail dot com.