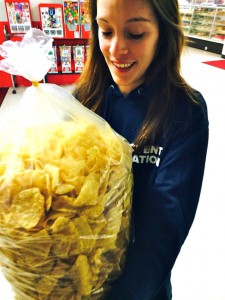
University of Texas at Austin grad student Dacia Leon (Twitter: @leondacia)
This post is by University of Texas at Austin grad student Dacia Leon (Twitter: @leondacia)
Fluorescence microplate readers are really exciting. These instruments are a staple in any synthetic biology lab given that they allow for high-throughput quantification of microbial growth and fluorescence over time – so many experiments, so much data. My lab recently purchased one of these microplate readers, and it rarely experiences the “OFF” state. A typical experimental setup consists of up to ninety-six engineered strains each containing a fluorescent reporter protein, which is commonly used as a proxy for expression of a synthetic device. As synthetic biologists, we use microbes as host organisms to “design-test-build” devices that are developed as a product of the researcher’s imagination. The nature of these synthetic devices varies greatly in that some address a pressing, societal need while others aim to explore the limits of biology. Our microplate reader is one way in which we can assay/troubleshoot our devices, and has therefore earned a valued position in our lab.
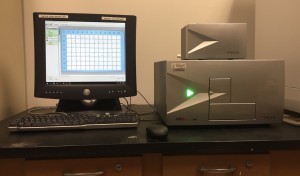
Our beloved microplate reader machine doing what it does best – taking time points every 15 minutes.
Reflecting on our enthusiasm for the microplate reader reminded me of a review, written over ten years ago discussing the fundamental principles of synthetic biology1. The primary author of the review, Drew Endy, states that one of the greatest limitations in engineering is that machines are built to be single-use. This means that once a machine is no longer functional, or simply antiquated, it is discarded or recycled. For example, our microplate reader is not designed to self-replicate and produce new generations of microplate readers, unfortunately. But, if given the possibility, would it not be incredible? Directions – incubating at 37°C overnight will yield hundreds of healthy, microplate reader colonies. The idea of self-replicating machines may seem absurd until we regard microbes as machines.
Genetic engineering of microbes has existed for decades, and there have been many successes in fields such as drug development, bioenergy, production of industrial chemicals, and agriculture. One of my favorite success stories is the biosynthesis of the antimalarial drug, artemisinin, in an engineered yeast host strain2. Artemisinin is naturally a plant-derived compound and its traditional method of isolation results in drastic fluxes in drug price and availability. Plant-based production of artemisinin consists of harvesting biomass from full-grown plants, which takes ~8 months, and then treatment with a solvent in order to extract the artemisinin. Unfortunately, this method is neither cheap nor stable and given the current death toll – over a million annually, most malaria patients are unable to access the treatment. To address this problem, a semi-synthetic artemisinin pathway was constructed in yeast to produce an artemisinin precursor, artemisinic acid, which can be chemically converted into artemisinin. The engineered yeast strain contained multiple modifications including three heterologous genes from the native artemisinin synthesis pathway and a series of alterations refining metabolic flux in the yeast host. Once synthesized, artemisinic acid is transported to the cell’s outer membrane, allowing for rapid purification, and subsequent conversion to artemisinin. Compared to the plant-based method, the engineered yeast host produces comparable levels of artemisinic acid, but over a markedly shorter time period (4-5 days) and using a yeast host omits the erratic nature of plant-based isolation from the process. Currently, research is focused on optimizing the industrial scale production of yeast artemisinin in order to advance it as a viable strategy against malaria.
There is one salient factor that I have excluded from this story so far. Microbes are replicating machines, but these machines are highly error-prone in their replication. Errors can stem from various sources such as environmental stress, the nature of the enzymes involved in DNA replication, and/or any toxic byproducts that are generated by a cell’s own metabolism. In any case, these replication errors lead to fixed mutations. Engineered microbes contain heterologous synthetic devices that utilize a heavy proportion of a cell’s resources for expression. These devices cause cellular stress and decrease fitness, resulting in a strong selection for mutations that eliminate expression of any synthetic part that comprises the device. Over time, random mutations that naturally occur in a living host organism will accumulate and render a synthetic device inactive. Microbes are limited by their inherent unpredictability. This poses a challenging problem for engineers.
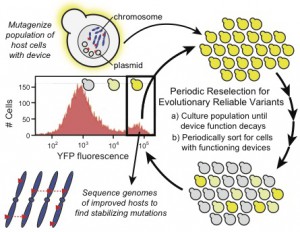
PResERV method work flow
To address this issue, one could engineer genetic stability on the side of the host organism, the synthetic device, or both. Synthetic devices can be re-designed by applying strategies such as removing repeat regions, optimizing codon usage, and altering gene expression. These strategies have been applied to device design and are shown to increase the lifetime of the device encoded in the host. One area that has been largely unexplored is on the side of the host organism. My work hypothesizes that the stability of the host genome can be significantly improved by decreasing the natural mutation rate of the host organism, resulting in a lower probability that the encoded synthetic device will mutate and become inactive. By lowering the baseline mutation rate, the host organism can be altered to accommodate any synthetic device, ensuring its long-term stability in the host. My goal is to engineer genetically stable host organisms and to understand the cellular mechanisms required for genetic stability. Our lab has developed an iterative, universal method called Periodic Reselection for Evolutionary Reliable Variants (PResERV), which enriches for genetically stable strains in a population using a fluorescent reporter gene. Mutants that maintain long-term expression of the synthetic fluorescent reporter gene are putative candidates with reduced mutation rates. These mutants are then isolated and sequenced to determine the causative mutations. PResERV will be used to identify genetically stable mutants in the two most commonly utilized host organisms, E. coli and yeast. By applying PResERV in both organisms, I will engineer reduced mutation variants and learn about relevant mechanisms in each organism.
Here are some of the questions I hope to answer with my research:
1.) How conserved are the cellular mechanisms that reduce mutation rates across species?2.) Can I develop general design principles for lowering mutation rates in any organism?3.) What is the limit to reducing mutation rates? Does this depend on the host organism?4.) How is the robustness of a reduced mutation host affected by increasingly complex synthetic devices?
Additionally, I think my work can provide knowledge and resources for the synthetic biology community. A collection of genetically stable host organisms will allow engineers to tackle more challenging problems without being limited by inactivating mutations.
References:
[1] Endy, D. Foundations for engineering biology. Nature 438, 449-453 (2005).
[2] Dae-Kyon R et al. Production of the antimalarial drug precursor artemisinic acid in engineered yeast. Nature 440, 940-943 (2006).